MTA-BME Lendület (Momentum)
Convolution Based Framework for Self-reliant Dynamic Description in Machining (CONWIB)
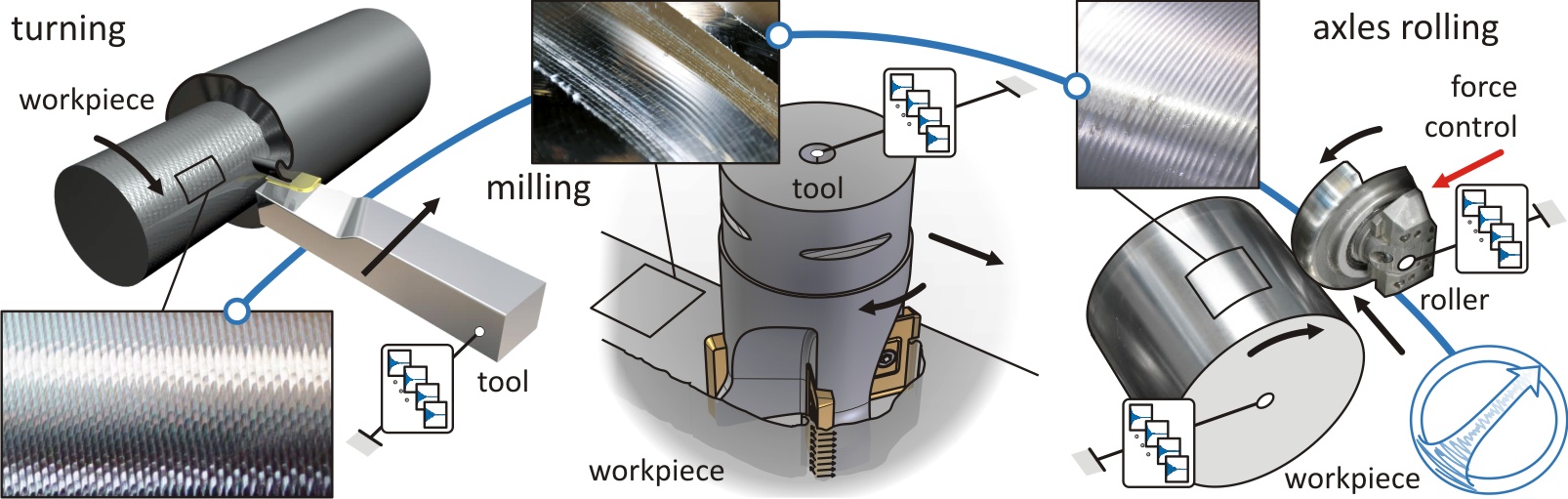
The 4th industrial revolution connects the automated and physical worlds via algorithm-driven environmental monitoring and intervention that increasingly augments human intelligence. Beyond the examples of our daily lives this progress also enables the manufacturing industry to enter into a new era. Versatile and advanced production capabilities require self-reliant process monitoring and predictive modelling to ensure faster fabrication with strict accuracy standards. Dynamics characterization and process modelling have relied on measurement techniques and the cumulative experience and knowledge of trusted experts. The project aims to revolutionize this methodology, by avoiding the construction of parameter-driven mathematical models and human-related interpretation of vibratory parameters. The concept applies convolution-based formalism of dynamical systems, where a kernel function replaces the role of vibratory parameters. The digital representation of the kernel can be considered as the digital image of the machine dynamics that is stored and compressed. The concept adapts methods of process modelling to the convolution framework by considering state, time and delayed state dependency. It is designed to serve as a self-reliant solution for modelling the varying dynamics of the workspace and the machined workpiece. This description is also introduced for machining related nonlinear phenomena by extending the linear kernel with rescaled compressed base. Nonlinearities cause load-dependent dynamics and nonlinear forcing, which modify the machine dynamics by changing its equilibrium, vibratory behavior, and by introducing special vibratory patterns. This breakthrough data-driven methodology is paired with high-gain techniques where the machine tool is aware of its dynamic capabilities, thus, its self-actuation is processed without any expert interaction to keep accuracy and avoid unstable (chatter) vibration for superior machining capabilities
The proposed concept aims to trigger a breakthrough in the vibration dynamics analysis procedure that development engineers use in machining industry. This industry naturally requires high precision in process prediction and control to ensure part accuracy, while increased production capabilities call for higher actuated motion speed that make machine tools more prone to vibrations. Computer science and information technology will radically change our daily routine in the near future by introducing autonomous vehicles, robotic surgery, etc. On the same basis, a 4th industrial revolution is expected [1] in the manufacturing sector introducing new IT services in factories for maximizing the production. The wave-front of this revolution is already approaching, however, the otherwise conservative manufacturing sector only introduces well established and reliable solutions due to their high responsibility in safety (airplanes, trains, etc. parts production). This new level of cyber-physical system ( CPS ) driven automation will revolutionise production in a way that machines can be operated safely and reliably in parameter ranges exceeding their passive limitations due to the intensive sensory and actuation interactions and interventions. To reach the highest maturity level, holonic [2] or, in other words, selfreliant and self-optimizing [1] CPS solutions are needed that are still a long way ahead. In manufacturing, cutting processes are directly influenced by the mechanical behaviour of the machine tool, which is critical to ensure accuracy and process stability [3]. Milling, turning, grinding and rolling are widely used to produce high-value parts that have ever increasing large demand worldwide like in the transportation industry with the gradual introduction of e.g. point-to-point air transit network and high-speed train lines. This CPS-driven world can only be achieved in process level by increasing the material removal rate, for which new generation of machine tools an additional high capacity real-time stability controller is also required to avoid unstable machining processes (chatter [4]) and to keep the accuracy standards. To achieve this level of automation, all three types of vibrations need to be handled. Free vibration is essential for dynamics characterization (e.g. hammer test [5]). Forced [6] and self-excited vibrations inevitably appear during cutting operations, nevertheless they both are completely ignored by conventional process planners ( CAM ). Both of the latter types are influenced by the regeneration (Figure 1, [7]) of the surfaces on the relatively rotating surface or/and edge segments of the tool and/or the workpiece [8]. The envisioned, continuous5 intervention must monitor the dynamic behaviour of the machine tool to eliminate selfexcitation and to decrease the effect of forced vibration, i.e. in order to avoid chatter and to keep accuracy. Moreover, there is a tendency in the machine tool industry to use versatile machine tools sometimes integrated with industrial robotic [9] solutions that are more prone to varying and nonlinear dynamic behaviour [10].
In order to intervene in the machining process efficiently, the sensory data must be processed autonomously producing effective actuation signals that decrease the relative vibration between the cutting edges and the workpiece. The underlying algorithm needs to have the on-line ‘complete picture’ of the mechanical properties and dynamic behaviour of the machine tool. Conventional methods used for the identification of the dynamics are based on expert interaction determining a point in the workspace without the mechanical load of the operation. These methods are off-line, time consuming, driven by subjective engineer expertise and lack the clear idea about the nonlinearities affecting the system. Existing tools for nonlinear analysis either serve too strict theoretical [11] frameworks, which are hard to implement and often fail, or provide solutions for well-prepared low dimensional special cases [12, 13] only.
Case Study:
Currently the contribution of a highly qualified expert to the analysis of machine tool vibrations is unavoidable when large amplitude vibrations occur during machining of high value parts with large size and/or intricate geometry. The vibrations can lead to reject parts, thus endanger a significant portion of the income and the good fame of a company. In a usual scenario, the expert travels to the site, performs measurements needed and gives recommendations according to his vast experience on dynamics identification and machine tool behaviour. The expert is able to extract vibratory parameters (such as natural frequency and damping), determine mode shapes with various resolution and positions, recognize nonlinear phenomena and qualitatively predict the machining process performance. The solutions for vibration attenuation [4] go beyond the simple changing of technological parameter(s) by designing and introducing passive [14] or semi-active dampers [15] on the machine structure.
The procedure described above is far from being objective and automated, although recent advances in the field of sensor and actuation techniques allow compact, fairly affordable and highly tailored embedded hardware solutions [16]. These tailor-made solutions enable the dynamics characterization of the machine, and reduce the need for significant intervention to the process, and doing so they substantially outperform the passive solutions. The challenge in this research lies in the replacement of the expert’s knowledge and experience. So far, the dynamics of the machines has never been described without human assistance focusing dominantly on mechanical modelling and on recognition of patterns in6 measurement data. The 4th industrial revolution will prevent such manual techniques in future autonomous machining applications.
References
- [1] Monostori L, Kádár K, Bauernhansl T, Kondoh S, Kumara S, Reinhart G, Sauer O, Schuh G, Sihn W, Ueda K (2016) Cyber-physical systems in manufacturing, CIRP Ann Manuf Techn, 65, 621-641.
- [2] Valckenaers P, Van Brussel H (2015) Design for the Unexpected, From Holonic Manufacturing Systems Towards a Humane Mechatronics Society, 1st ed. Elsevier.
- [3] Altintas Y (2012) Manufacturing Automation: Metal Cutting Mechanics, Machine Tool Vibrations, and CNC Design, Cambridge University Press.
- [4] Munoa J, Beudaert X, Dombovari Z, Altintas Y, Budak E, Brecher B, Stepan G (2016) Chatter suppression techniques in metal cutting, CIRP Ann Manuf Techn, 65, 785-808.
- [5] Dombovari Z (2017) Dominant modal decomposition method, J Sound Vib, 392, 56-69.
- [6] Bachrathy D, Insperger T, Stepan G (2009) Surface properties of the machined workpiece for helical mills, Mach Sci Technol, 13, 227-245.
- [7] Stepan G (1989) Retarded dynamical systems, London, Longman.
- [8] Dombovari Z, Stepan G (2015) On the bistable zone of milling processes, Phil Trans R Soc A, 373, 20140409.
- [9] Ozturk E, Barrios C, Sun C, Rajabi S, Munoa J (2018) Robotic assisted milling for increased productivity, CIRP J Manuf Sci Techn, 67, 427-430.
- [10] Wernholt E, Gunnarsson S (2008) Estimation of nonlinear effects in frequency domain identification of industrial robots, IEEE T Instrum Meas, 57, 856-863.
- [11] Breunung T, Haller G (2018) Explicit backbone curves from spectral submanifolds of forced-damped nonlinear mechanical systems, P Roy Soc A-Math Phy, 474, 20180083.
- [12] Gifford S J, Tomlinson, G R (1989) Recent advances in the application of functional series to non-linear structures, J Sound Vib, 135, 289-317.
- [13] Kerschen G, Worden K, Vakakis A F, Golinval J C (2006) Past, present and future of nonlinear system identification in structural dynamics, Mech Syst Signal Pr, 20, 505-592.
- [14] Sims N D (2007) Vibration absorbers for chatter suppression: a new analytical tuning methodology, J Sound Vib, 301, 592-607.
- [15] Munoa J, Iglesias A, Olarra A, Dombovari Z, Zatarain M, Stepan G (2016) Design of self-tuneable mass damper for modular fixturing systems, CIRP J Manuf Sci Techn, 65, 389-392.
- [16] EU FP7 DYNXPERTS (FP7-NMP-ICT-Fof-260073)
Other Important Literature
- Altintas 2000 Manufacturing Automation
- Tlusty 1982 CIRP Annals 31(1) 195-199
- Yang 2007 ASME J Dyn Sys Measr Contr 134(4) 041001
- Altintas et al. 2004 CIRP Annals 53(2) 619-642
- Monostori et al. 2016 CIRP Annals 65(2) 621-641
- Gilchrist 2016 Industry 4.0. 195-215
- Bernardo 2007 Piecewise-smooth Dynamical Systems
- Haylen et al. 2007 Modal Analysis Theory and Testing
- Guckenheimer et al. 1983 Nonlinear Oscillations
- Ewins 2000 Modal Testing: theory, practice, and applications